Wastewater: Our essential guide to wastewater treatment, management & solutions
Table of contents
- Why is wastewater treatment important?
- What is wastewater treatment?
- Wastewater pre-treatment (preliminary treatment)
- Three stages to wastewater treatment process
- 4 Advanced waste water solutions
- Wastewater recovery
- Industrial Wastewater Treatment
Image credit: World Bank Group
Wastewater treatment, collection, and discharge are essential for protecting human health, the environment, and water quality. Before treatment, wastewater must be collected from sewer networks servicing homes, municipal, commercial, and industrial premises, including rainwater run-off from roads and impermeable surfaces. Wastewater and industrial wastewater treatment are evolving. Initially focused on cleaning before safe discharge, today, wastewater is recognized as a valuable resource for energy, nutrients, and water.
This article covers the various treatment stages and technologies in wastewater treatment.
Wastewater: Creating sustainable value
Why is wastewater treatment important?
Today, around 80% of global wastewater is discharged into waterways, posing health, environmental, and climate hazards, as reported by the IWA. Wastewater treatment capacity stands at 70% in high-income countries but only 8% in low-income countries.
Urbanization intensifies this challenge, increasing wastewater generation and depleting Earth's resources, according to the IWA. Untreated effluent discharge contributes to eutrophication, human health risks, and substantial Greenhouse Gas (GHG) emissions, including nitrous oxide and methane. Emissions from untreated sewage are three times that of conventional wastewater treatment.
Even with poor treatment coverage in emerging cities, emissions from untreated sewage can constitute a significant percentage of cities' global emissions. Wastewater management and sewer systems are crucial for sanitation and disease prevention, preventing contamination of the local environment and drinking water supply, thereby reducing the risk of disease transmission.
lobal access to safe water, sanitation, and hygiene education is vital for reducing disease, improving health, and fostering socio-economic development. Unfortunately, in many countries, challenges such as limited resources, investment, infrastructure, technology, and space hinder proper wastewater management. This leaves populations at risk of water, sanitation, and hygiene (WASH)-related diseases.
The 2030 Agenda for Sustainable Development, specifically Goal 6, aims to ensure sustainable water and sanitation management for all by 2030. However, as of 2015, 2.1 billion people lacked access to safe drinking water, and 4.5 billion, or six in ten, lacked safely managed sanitation facilities.
Beyond preserving human health and environmental well-being, modern wastewater treatment plays a crucial role in identifying ways to extract value from materials, energy, and water embedded in wastewater streams.
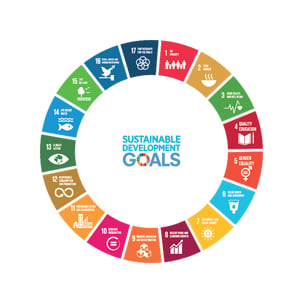
Image credit: ILO
What is wastewater treatment?
Wastewater treatment involves extensive sewer networks spanning thousands of kilometres, varying in size across regions and countries. In the UK, the largest systems link to approx. 9000 wastewater treatment plants.
Some areas use separate surface water sewers for rain, directing it into rivers. In contrast, others combine wastewater and surface water in sewers before treatment, demonstrating wastewater management's adaptability to local conditions.
In the UK, recent fibre sensing cable developments assess flow, depth, temperature, and structural integrity every five meters along sewer pipes.
Once wastewater reaches treatment plants, four stages include:
- Preliminary treatment (pre-treatment): Removes grit, gravel, and screens large solids.
- Primary treatment: Settles larger suspended organic matter.
- Secondary treatment: Biologically breaks down and reduces residual organic matter.
- Tertiary treatment: Addresses various pollutants using diverse treatment processes.
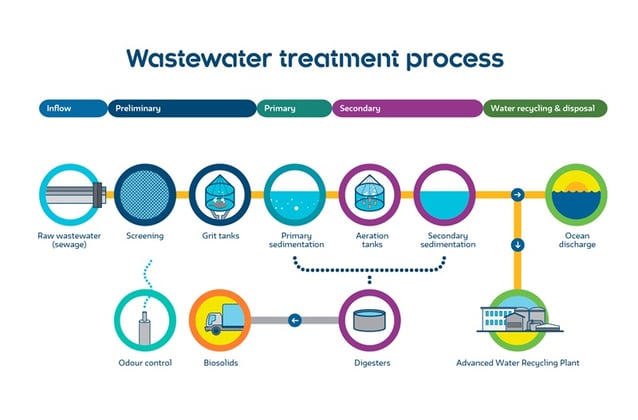
Image credit: Water Corporation
In wastewater treatment, a mixture of solids and water, known as sludge, is produced. The IWA notes that the volume of sludge generated in a WWTP is only about 1% (0.5% when dewatered) of the influent wastewater volume. Efficient WWTP management requires the extraction of waste sludge, including inert solids and excess biomass, to prevent system accumulation.
In a WWTP, the types of sludge produced are:
- Primary sludge is generated from settleable solids removed in the initial settling of raw wastewater. It exhibits high putrescibility, good dewaterability compared to biological sludge, with total solids content ranging from 2-5%.
- Secondary sludge, also known as biologica sludge, results from biological processes like activated sludge or biofilm systems. It comprises microorganisms grown on biodegradable matter, endogenous residue, and inert solids not removed in primary settling. Total solids content in secondary sludge ranges from 0.5-1.5%.
- Chemical sludge is formed through the precipitation of specific substances (e.g., phosphorus) or suspended solids.
Wastewater pre-treatment (preliminary treatment)
Pre-treatment plays a pivotal role in the holistic efficiency of wastewater treatment processes by acting as a frontline defense against potential interferences. Its critical significance extends to protecting the integrity of raw water lifting systems, pipelines, and various treatment equipment, mitigating the risk of blockages and abrasion. The overarching objective is to eliminate any factors that might impede the effectiveness of subsequent treatment, ultimately contributing to the prolonged lifespan of sanitation infrastructure and diminishing the wear and tear on mechanical components.
The spectrum of pre-treatment operations is diverse, providing wastewater treatment plants with a range of options adaptable to the unique characteristics of raw water. These operations encompass bar screening, straining, comminution, grit removal, grease removal (often in tandem with grit removal), oil removal, and the treatment of by-products such as grit and grease. Additionally, some plants may opt for the combined treatment of mains cleaning waste and plant grit.
While the advantages of pre-treatment are evident in its relatively low capital and operational costs, as well as its reduced risk of adversely affecting subsequent conveyance and treatment technologies, it's essential to acknowledge a notable drawback. The efficacy of pre-treatment processes requires frequent maintenance to ensure sustained effectiveness, underscoring the ongoing commitment required for optimal functionality.
Moving to the initial unit operation in wastewater treatment plants, screening acts as a crucial gateway to maintaining operational integrity. It serves the purpose of eliminating objects that could potentially cause damage and blockages in downstream equipment and piping. This process, incorporating both coarse and fine screens depending on the plant's modernity, establishes an early defense mechanism against undesirable materials.
Coarse screens, characterized by openings of 6 mm (0.25 in) or larger, efficiently remove large solids, rags, and debris from wastewater. Mechanically and manually cleaned bar screens, along with trash racks, are integral components of this screening process. In contrast, fine screens, with typical opening sizes ranging from 1.5 to 6 mm (0.06 to 0.25 in), prove instrumental in removing materials that might pose operational and maintenance challenges downstream, particularly in systems without primary treatment.
The strategic utilization of very fine screens, featuring openings of 0.2 to 1.5 mm (0.01 to 0.06 in) after coarse or fine screens, adds an additional layer of refinement. This advanced screening approach further reduces suspended solids to levels comparable to those achieved by primary clarification, showcasing a nuanced yet impactful method of ensuring the comprehensive removal of undesirable materials. This meticulous screening strategy plays a pivotal role in enhancing the overall efficiency and effectiveness of wastewater treatment processes.
Image credit: Sustainable Sanitation and Water Management Toolbox
Three stages to wastewater treatment process
Wastewater treatment is a multifaceted process crucial for maintaining environmental health and safety. The treatment involves a sequence of stages, each with distinct objectives and methods to ensure the effective removal of pollutants.
n this comprehensive overview, we delve into the three key phases of wastewater treatment: primary, secondary, and tertiary. Each stage contributes uniquely to the overall purification process, addressing specific contaminants and enhancing the quality of treated water.
From the initial removal of settleable solids in primary treatment to the advanced processes of tertiary treatment, this exploration sheds light on the intricate mechanisms that safeguard water resources and promote sustainable environmental practices.
Image credit: Accutherm
1. Primary treatment
The objective of primary treatment is the removal of settleable organic and inorganic solids by sedimentation, and the removal of materials that will float (scum) by skimming. Approximately 25% to 35% of the incoming biochemical oxygen demand (BOD), 50 to 70% of the total suspended solids (SS), and 65% of the oil and grease are removed during primary treatment. Some organic nitrogen, organic phosphorus, and heavy metals associated with solids are also removed during primary sedimentation but colloidal and dissolved constituents are not affected.
2. Secondary treatment
Secondary treatment, typically following primary treatment, removes residual organics and suspended solids. This process entails eliminating biodegradable dissolved and colloidal organic matter through aerobic biological treatment. Aerobic microorganisms, mainly bacteria, metabolize the organic matter in the wastewater in the presence of oxygen, generating more microorganisms and inorganic end-products.
Various aerobic biological processes are employed for secondary treatment, differing in oxygen supply and organism metabolization rates. Common high-rate processes include activated sludge trickling filters or biofilters, oxidation ditches, and rotating biological contactors, collectively contributing to achieving the goal of secondary treatment.
In the activated sludge process, the dispersed-growth reactor is an aeration tank containing wastewater and microorganisms, creating a mixed liquor. Aeration devices, like submerged diffusers and mechanical surface aerators, mix the tank vigorously and supply oxygen to the biological suspension. The hydraulic retention time in aeration tanks usually ranges from three to eight hours, but it can be higher with high BOD wastewaters.
After aeration, microorganisms are separated from the liquid by sedimentation, producing clarified liquid as secondary effluent. Part of the biological sludge is recycled as return activated sludge (RAS) to maintain a high mixed-liquor suspended solids (MLSS) level. The remaining sludge (SAS) or waste activated sludge (WAS), is removed for processing, ensuring a relatively constant microorganism concentration in the system.
3. Tertiary treatment
Tertiary and/or advanced wastewater treatment is essential for removing specific constituents resistant to secondary treatment. Targeting nitrogen, phosphorus, suspended solids, refractory organics, heavy metals, and dissolved solids, individual treatment processes are utilized. These advanced methods seamlessly integrate with primary or secondary treatment, as seen in chemical additions to primary clarifiers or aeration basins to remove phosphorus. In some cases, advanced treatments replace traditional secondary methods, such as overland flow treatment of primary effluent, ensuring a comprehensive approach to enhance water quality.
4 Advanced wastewater solutions
Various advanced treatment solutions have emerged in response to the demand for enhanced operational cost efficiency (OPEX), reduced plant footprints, and stricter discharge regulations. Here, we emphasize four pivotal wastewater solutions.
1. Moving Bed Biofilm Reaction (MBBR) Technology
MBBR technology utilizes numerous polyethylene biofilm carriers, which move in a mixed motion within an aerated wastewater treatment basin.
Each biocarrier enhances productivity by offering a protected surface area for the growth of heterotrophic and autotrophic bacteria within its cells. This high-density population of bacteria facilitates high-rate biodegradation within the system.
MBBR processes can self-maintain an optimum level of productive biofilm which, when attached to the mobile biocarriers within the system automatically responds to load fluctuations, according to Headworks International.
Image credit: Gustawater
2. Membrane Bioreactors (MBR)
“Membrane Bioreactor” (MBR) combines a perm-selective membrane (e.g., microfiltration or ultrafiltration) with a suspended growth bioreactor in wastewater treatment. In contrast to “polishing” processes, MBRs integrate the membrane into the biological treatment directly.
Image credit: SUEZ water handbook
3. Membrane aerated biofilm reactor (MABR)MABR systems passively circulate oxygen through a spirally wound membrane at atmospheric pressure. MABR’s self-respiring membrane allows bacteria to consume oxygen more readily for a 90% reduction in energy used for aeration.
Most commercial MBR processes use the membrane as a filter, resulting in clarified and disinfected effluent. This integrated approach ensures effective contaminant removal for a streamlined wastewater treatment solution.
The membrane surface accumulates a biofilm of bacteria that establishes a simultaneous nitrification-denitrification (SND) process to produce a high-quality, low-nitrogen effluent suitable for reuse in irrigation.
Image credit: Blower & Vacuum Best Practices
4. Ultraviolet (UV)
Image credit: Environmental Science & Engineering Magazine
For advanced wastewater treatment plants, ultraviolet (UV) technology has been included in the tertiary treatment process. This can allow the wastewater treatment plant to meet even more stringent requirement, in some cases for indirect and direct potable reuse and water reclamation.
The wavelengths of UV light range between 200 and 300 nanometers (billionths of a meter). Special low-pressure mercury vapor lamps produce ultraviolet radiation at 254 nm, the optimal wavelength for disinfection and ozone destruction. Categorised as germicidal, this means they are capable of inactivating microorganisms, such as bacteria, viruses and protozoa. The UV lamp never contacts the water; it is either housed in a quartz glass sleeve inside the water chamber or mounted external to the water which flows through UV transparent Teflon tubes.
One notable development to UV systems is the scaling up of light-emitting diode technology, known as UV-LED, with 2018 witnessing a tipping point on power density and purchasing price.
Wastewater recovery
Water reuse is a form of wastewater recovery whereby water can be extracted for purposes such as agricultural and golf course irrigation, rather than being discharged to the environment. This ties in with a growing trend to see wastewater treatment plants instead as resource recovery centres. Recovering the water, energy, nutrients and other precious materials embedded in wastewater is a key opportunity, according to the IWA.
Image credit: World Bank Group
Used water is one of the most under-exploited resources available. Water from industrial or domestic use contains energy, water, organics, phosphates, nitrogen, cellulose, rare earths, and other resources. The IWA said technologies are increasingly making resource recovery from wastewater commercially feasible, including bio-gas, fertiliser, paper, metals, plastics and, perhaps most importantly, it is a source of ‘new’ water.
Industrial Wastewater Treatment
Most water reuse applications prior to the last decade were producing secondary quality water for industrial or agricultural purposes. These will still provide major uses for lower grade reused wastewater. However, for potable and some industrial purposes, a high level of treatment is required.
When addressing the question of why reuse wastewater, one answer is because you've already paid for the treatment so why not make the most of this resource.
Techniques for potable water reuse caninvolve membrane-based techniques such as ultrafiltration (UF) and reverse osmosis (RO), and using ultraviolet (UV) light or ozone for disinfection. Lately, these are finding other applications in industry. Other techniques such as electrodialysis, ceramic membranes and advanced oxidation are also being used in novel ways to enable wastewater reuse.
For potable purposes, the industry has split wastewater reuse into indirect (IPR) and direct (DPR) reuse, the latter requiring much more stringent standards and approvals than the former.
The city-state of Singapore has long been the pathfinder in reusing wastewater for potable purposes - NEWater, as the government terms it. Yet the small cities of Big Spring and Wichita Falls in Texas and the much larger city of San Diego in California will probably be better remembered as ushering in DPR across the world.
Image credit: Orange County Water District
The US already hosts a world-leading example of IPR in the Orange County Groundwater Replenishment System in California. Meanwhile, Australia boasts an equally large project, the 232,000 m3/day Western Corridor Recycled Water Project in Queensland. This has three advanced wastewater treatment plants, which contribute reused water to industry and agriculture.
Share your water technology stories with us
Do you have an innovation, research results or an other interesting topic you would like to share with the international water technology industry? The Aquatech website and social media channels are a great platform to showcase your stories!
Please contact our Sr Brand Marketing Manager Annelie Koomen.
Are you an Aquatech exhibitor?
Make sure you add your latest press releases to your Company Profile in the Exhibitor Portal for free exposure.
We promise never to send you spam and you can unsubscribe at any time!